Industrial waste is produced as a residue of production systems. Manufacturing products in industries generate a range of unwanted by-products disposed of in water or landfill spaces. World’s Stats (2024) shows that 1,300 million metric tons of industrial waste is generated annually. This waste disposal poses multiple environmental risks, such as pollution, biodiversity loss, ecosystem destruction, and climatic changes.
Recycling metals is crucial for conserving resources and energy by reducing energy consumption. Just by recycling aluminum, we can “save 95% of the energy used in its primary production” (Stena Recycling, n.d.). Similarly, estimated energy saved is 90% for copper recycling and 60% for iron and steel recycling (Recon Metal, n.d.).
Extracting metals from waste reduces its environmental footprint by minimizing CO2 emissions and the need for mining. Many physical, chemical, and thermal methods are used to extract metals, namely acid leaching, open burning, mercury amalgamation, etc. (see, for example, Krishnan et al., 2021).
However, as most of these are unsustainable and costly and rely on expensive equipment, bioleaching offers a more viable approach. Bioleaching uses microorganisms to extract metals, and is applicable to low-grade waste, reducing chemical usage, energy consumption, and residue generation (Srichandan et al., 2019).
Bioleaching for Metal Extraction
With the increase in urbanization, the rapid growth of industrial activities has resulted in the generation of massive amounts of waste (Voukkali et al., 2023). Industrial waste contamination into soil, water, and air severely affects ecosystems and human health. Health issues can occur through ingestion, inhalation, and skin contact with industrial waste.
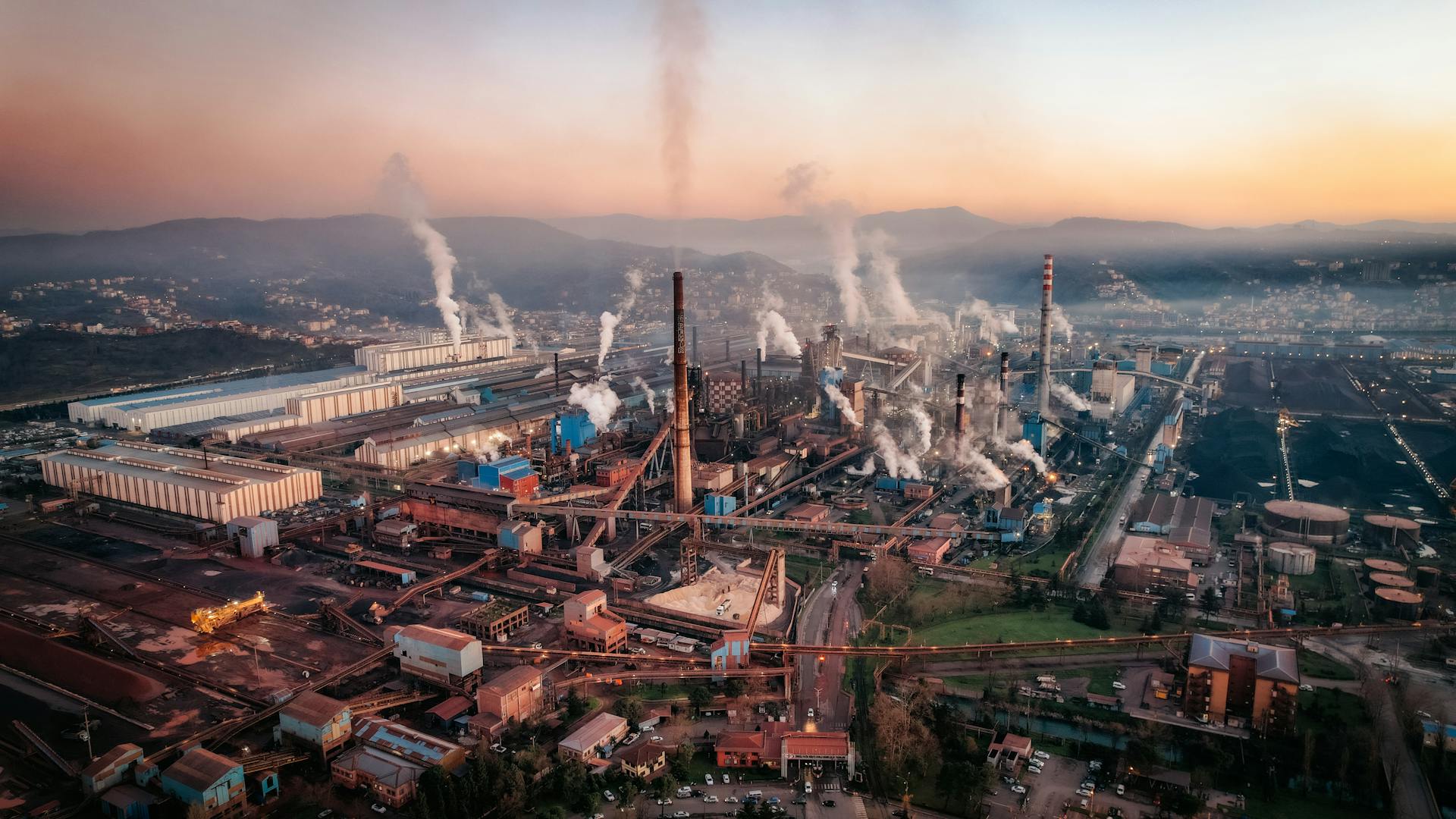
Waste can be characterized as hazardous and non-hazardous waste. Hazardous waste contains toxins, while non-hazardous waste, although not toxic, is detrimental to the environment in other ways (Awuchi et al., 2020). Industrial by-products can come from multiple sources, including mining industries, manufacturing industries, construction, refining, or demolition activities. Metals, in addition to being carcinogenic (cancer causing), also have the properties to cause neurogenic disorders, organ damage, and congenital disabilities (Zhu & Costa, 2020).
Several methods are used to extract metals from waste. The effective conventional methods are listed below.
- Pyrometallurgy: using elevated temperatures for extraction in processes like smelting.
- Electrometallurgy: using high-intensity electric current for extraction.
- Mechanical separation: crushing and grinding waste to separate metals from waste.
Bioleaching is innovative method of extracting metals using microorganisms. The basic principle is that microorganisms break down metal-bearing waste to release metals into the solution (Bosecker, 1997). This is done through the formation of a metal-ligand complex by the metal ion and the said microorganism (acting as a ligand). The mechanism of complex formation can be divided into four major steps: attachment, oxidation, complexation, and solubilization.
Microorganisms attach themselves to the waste minerals and then oxidize the residue, releasing metals into the solution. The microorganism’s complex formation with the metal ions enhances the metals’ solubility, efficiently extracting them from the solution (Mishra et al., 2005).
Bioleaching is used because of its multiple environmental, economic, and social benefits compared to traditional methods. One is the reduction of the mass of waste deposited in landfills, thereby decreasing pollution. It is also more cost-effective as this technique can be applied to low-quality ores. Another reason bioleaching is a cost-effective process is because there is no high energy requirement or use of expensive chemicals.
Compared to conventional extraction methods, bioleaching operates in milder conditions can be scaled up or down based on the application (Saldaña et al., 2023). This technique is also more selective and can extract specific materials, reducing waste generation.
Diverse types of micro-organisms are used for the extraction of metals; commonly used ones are bacterium Chromobacterium violaceum, Pseudomonas fluorescens, and Leptospirillum used in the bioleaching of Copper, Gold, Nickel, Cobalt, and Uranium (Brandl et al., 2008). Fungus used in bioleaching is either Aspergillus Niger or Penicillium Simplicissimum for the extraction of Copper, Zinc, and Lead (Schinner & Burgstaller, 1989). These microorganisms work on waste treatment through direct and indirect leaching (Mishra et al., 2005). They either oxidize the waste directly or produce compounds that oxidize metal-containing waste (indirect leaching).
Current Trends and Challenges
Bioleaching has been applied in multiple industries to date. Mining and medical waste have often been treated with microorganisms to extract metals. Bioleaching can also extract metals from spent catalysts and platting and deposition industries.
Removal of contaminants (such as heavy metals) by living or non-living biomass (organic matter derived from living things) is called biosorption. In a study treating Rungkut Industrial Sewage in Indonesia, bacteria and yeast were examined to biosorb copper metal from industrial sewage. This report predicted the use of microorganisms in a cyclic economy by wastewater treatment (Irawati et al., 2017).
Mishra (2022) describes zinc metal extraction from industrial waste using a Penicillium sp. fungus that produces citric acid as the leaching agent. Furthermore, cyanide-producing microorganisms are used to complex gold and recover from waste (Maluckov, 2021). Likewise, silver and platinum can also be extracted using cyanogenic microorganisms (Brandl et al., 2008).
The choice of microorganisms depends on the application, as industrial waste can be toxic and inhibit their growth (Yaashikaa et al., 2022). Scalability and commercialization are also challenges requiring significant investment. As this is a comparatively new extraction method, a regulatory framework is hard to achieve (Petersen, 2023).
Future research directions are using genetic technology to enhance the resistance and metal extraction capabilities of microorganisms (Pattanaik et al., 2020). The development of an efficient bioleaching reactor can scale up the extraction. In addition, machine learning and artificial intelligence tools can predict microorganism resistance, degree of complexation, and microbial performance.
As the world faces a waste management crisis, it is integral to adopt sustainable practices that move towards a cyclic economy. Research and development of extraction methods focusing on bioleaching can prove fruitful.
Industries should be encouraged to adopt eco-friendly metal extraction processes, including collaboration between companies and the public sector in treating industrial waste with metal-mobilizing microorganisms.
References
Awuchi, C. G., Hannington, T., Awuchi, C. G., Igwe, V. S., & Amagwula, I. O. (2020). Industrial waste management, treatment, and health issues: wastewater, solid, and electronic wastes. European Academic Research, 8(2), 1081-1119.
Bosecker, K. (1997). Bioleaching: metal solubilization by microorganisms. FEMS Microbiology reviews, 20(3-4), 591-604.
Brandl, H., Lehmann, S., Faramarzi, M. A., & Martinelli, D. (2008). Biomobilization of silver, gold, and platinum from solid waste materials by HCN-forming microorganisms. Hydrometallurgy, 94(1-4), 14-17.
Irawati, W., Parhusip, A. J., Christian, S., & Yuwono, T. (2017). The potential capability of bacteria and yeast strains isolated from Rungkut Industrial Sewage in Indonesia as a bioaccumulators and biosorbents of copper. Biodiversitas Journal of Biological Diversity, 18(3), 971-977.
Krishnan, S., Zulkapli, N. S., Kamyab, H., Taib, S. M., Din, M. F. B. M., Abd Majid, Z., … & Othman, N. (2021). Current technologies for recovery of metals from industrial wastes: An overview. Environmental Technology & Innovation, 22, 101525.
Maluckov, B. S. (2021). Biorecovery of nanogold and nanogold compounds from gold-containing ores and industrial wastes. Applied Microbiology and Biotechnology, 105(6), 3471–3484.
Mishra, D., Kim, D. J., Ahn, J. G., & Rhee, Y. H. (2005). Bioleaching: a microbial process of metal recovery; a review. Metals and Materials International, 11, 249-256.
Mishra, S. P. (2022). Removal of heavy metal ions from copper and zinc industrial effluents using Penicillium sp. International Journal of Environmental Science and Technology, 19(9), 9107-9114.
Pattanaik, A., Samal, D. K., Sukla, L. B., & Pradhan, D. (2020). Advancements and use of omic technologies in the field of bioleaching: a review. Biointerface Res Appl Chem, 11, 10185-10204.
Petersen, J. (2023). From understanding the rate limitations of bioleaching mechanisms to improved bioleach process design. Hydrometallurgy, 221, 106148.
Saldaña, M., Jeldres, M., Galleguillos Madrid, F. M., Gallegos, S., Salazar, I., Robles, P., & Toro, N. (2023). Bioleaching modeling–a review. Materials, 16(10), 3812.
Schinner F, & Burgstaller W. (1989). Extraction of Zinc from Industrial Waste by a Penicillium sp. Appl Environ Microbiol 55(5), 1153 – 1156.
Srichandan, H., Mohapatra, R. K., Parhi, P. K., & Mishra, S. (2019). Bioleaching approach for extraction of metal values from secondary solid wastes: a critical review. Hydrometallurgy, 189, 105122.
Voukkali, I., Papamichael, I., Loizia, P., & Zorpas, A. A. (2023). Sustainable waste management & circular economy: Urbanization and solid waste production: Prospects and challenges. Environmental Science and Pollution Research, 30, 61153–61166.
Yaashikaa, P. R., Priyanka, B., Kumar, P. S., Karishma, S., Jeevanantham, S., & Indraganti, S. (2022). A review on recent advancements in recovery of valuable and toxic metals from e-waste using bioleaching approach. Chemosphere, 287, 132230.
Zhu, Y., & Costa, M. (2020). Metals and molecular carcinogenesis. Carcinogenesis, 41(9), 1161-1172.